Translate this page into:
A Clinical Approach to Diffusion-Weighted Magnetic Resonance Imaging in Evaluating Chest Wall Invasion of Breast Tumors
* Corresponding author: Dr. Naziya Samreen, Department of Radiology, Mayo Clinic, 200 First St. SW, Rochester 55905, MN, USA. samreen.naziya@gmail.com
-
Received: ,
Accepted: ,
Abstract
Objective:
The purpose of this study is to evaluate diffusion weighted magnetic rsonance imaging (MRI) acquisitions in delineating posterior extent of breast tumors and in predicting chest wall invasion prior to treatment. To our knowledge, there has not been any literature specifically evaluating the utility of diffusion-weighted acquisitions in chest wall invasion of breast tumors.
Materials and Methods:
A retrospective review of our breast imaging database for keywords “chest wall invasion” and “breast MRI” was performed over the last 14 years. Diffusion sequences, T1 sequences (pre and post contrast), and T2 sequences were evaluated. Apparent diffusion coefficient (ADC) values in tumor and chest wall were assessed. Imaging findings were correlated with surgical pathology.
Results:
23 patients met inclusion criteria. All 23 had loss of fat plane on T2 sequences. 22/23 had loss of fat plane on postcontrast T1 sequences. Pectoralis muscle enhancement was present in 19/23 (83%) tumors and chest wall enhancement was present 9/23 (39%) tumors. Qualitative restricted diffusion within the pectoralis muscle was present in 18/23 (71%) tumors and in the chest wall was present in 8/23 (35%) tumors. Mean ADC values were 1.15 s/mm2 in the tumor and 1.29 s/mm2 in the chest wall. Sensitivity, specificity, positive predictive value and negative predictive value were 100%, 36%, 63%, and 100% for chest wall enhancement respectively and 69%, 36%, 61%, and 80% for chest wall diffusion-weighted imaging restriction respectively.
Conclusion:
Diffusion weighted sequences can be helpful in characterizing chest wall invasion of breast tumors.
Keywords
Breast cancer
breast tumors
chest wall invasion
diffusion weighted magnetic resonance imaging
INTRODUCTION
According to the American Joint Committee on Cancer staging guidelines for breast cancer, tumor of any size with direct extension to the chest wall is classified as T4 disease[1] which carries a poor prognosis. Chest wall invasion includes direct invasion of ribs, serratus anterior muscle, or intercostal muscles. Involvement of the pectoralis major or minor muscles alone is not considered chest wall involvement and hence does not by itself change clinical breast cancer staging, but it does alter surgical management.
Imaging assessment for malignant chest wall extension can be challenging.
Mammographically, it may be technically prohibitive to include such far posterior locations in the field of view, and ultrasonographically, it may be difficult to identify a normal fat plane interface between the tumor and the pectoralis muscle. On the other hand, breast magnetic resonance imaging (MRI) is particularly helpful for staging and preoperative evaluation in patients with posterior breast tumors.
A study by Morris et al., showed that chest wall enhancement on MRI correlated well with tumor invasion at surgery[2] and was more specific when compared to loss of fat plane on MRI. Another MRI technique called diffusion weighted imaging (DWI) is based on differences in Brownian motion of water molecules and is often used to distinguish normal from abnormal tissues.[3-9] DWI techniques most frequently use spin-echo echo-planar imaging pulse sequences to reflect the T2* differences between diffusion capabilities of water molecules in differing local environments. Diffusion gradients applied on either side of a 180° pulse can be tailored to various degrees of diffusion. The user-defined b-value instantiates the necessary gradient characteristics so that the higher the b-value, the greater the signal attenuation due to diffusion. This means that water molecules that experience restricted diffusion, such as those in neoplastic processes with increased cellularity, have higher signal on DWI. Diffusion, however, is multi-directional, so DWI is usually performed with four acquisitions, one at b = 0 s/mm2and three more to account for the x, y, and z directions at a user-specified b-value. Subsequent mathematically-derived isotropic DWI which lacks directionality is used for interpretation, and calculated metrics called apparent diffusion coefficients (ADC) quantitatively reflect the extent of restricted diffusion.[10,11] On breast MRI, prior studies have shown that malignant breast lesions show more diffusion restriction compared to benign breast lesions.[6-8,10,12-16]
In the last decade, various DWI techniques have been developed to address its relative low resolution, susceptibility to distortion, T2 effects, field-strength effects, and accuracy in ADC calculations.[6-9,11] Such variations make it challenging to rigorously investigate DWI. Nevertheless, DWI plays a major role in oncologic imaging, particularly for assessing extent of disease.[17-20] The purpose of this study is to describe how DWI is used at one institution to assess chest wall invasion of breast tumors which underwent neoadjuvant chemotherapy (NAC) and to compare it with conventional MRI evaluation and pathology.
MATERIALS AND METHODS
A retrospective search of the radiology report database using keywords “chest wall invasion” and “breast MRI” at our institution between 2003 and 2017 yielded 58 such cases. MRIs without DWI acquisitions or unassociated with staging prior to NAC or surgery were excluded. Using these exclusion criteria, thirty cases were excluded. In addition, 5 of the 28 patients were lost to follow-up after initial diagnosis, and these 5 were excluded from analysis. The remaining 23 breast MRI cases were evaluated in this investigation.
Magnetic resonance imaging technique
All 28 breast MRIs were performed at 1.5 Tesla using a dedicated bilateral breast coil (GE, Boston, MA). All studies met criteria for breast MRI accreditation set forth by the American College of Radiology. Water and fat axial images were acquired from a T2-weighted fast spin-echo acquisition using a modified 3-point Dixon (IDEAL or Iterative Decomposition of water and fat with Echo Asymmetry and Least-squares estimation) method for fat suppression, field of view 28-40 cm, slice thickness 4.0 mm with 1.0 mm spacing, TR/TE 4505 ms/102 ms, echo-train length 14, matrix 320 × 224, NEX 2.0, and bandwidth 50.0 kHz. Axial, fat-suppressed pre-gadolinium and post-gadolinium 3D spoiled gradient-recalled (SPGR) images were acquired at 1–1.5 min, 2–3 min, and about 7–8 min after intravenous injection of gadolinium-based contrast agent (0.1 mmol/kg) using field of view 28–40 cm, slice thickness 1.8–2.0 mm, in-plane resolution ≤1.0 mm, TR/TE 5.2 ms/2.5 ms, flip angle 10°, bandwidth 62.50 kHz, and phase direction LR. Between 3 and 7 min after contrast injection, a higher-resolution axial 3D SPGR image was acquired with field of view 28-40 cm, slice thickness 1.2–1.4 mm, in-plane resolution ≤1.0 mm, TR/TE 5.2 ms/2.5 ms, bandwidth 62.50 kHz, and phase direction AP.
All DWI images were acquired using a spin-echo echo-planar-imaging pulse sequence with b values 0, 800 s/mm2, TR/TE 4000 ms/minimum, slice thickness 5 mm, slice spacing 1 mm, and acquisition matrix 128 × 128 in the axial or sagittal planes.
Qualitative radiological assessment
Diagnostic interpretation for presence of chest wall invasion based on T2-weighted, DWI, and dynamic contrast-enhanced (CE) acquisitions was then performed through consensus readings by two board-certified breast imagers (C. L. and K. G.). All breast MRI cases were viewed using the Aegis Breast imaging software (Hologic, Inc Marlborough, MA) which automatically generates ADC values. Radiological assessment of posterior extent of disease was categorized into: loss of the posterior fat plane, involvement of the chest wall (strictly the ribs, intercostal muscles, or serratus anterior muscle), and involvement of the pectoralis muscle. Each category was evaluated using T2-weighted, CE, and DWI. Presence of a particular area of anatomic involvement was evaluated independent of other anatomic areas of involvement; therefore, a single case may have more than one area of involvement. Appreciable differences were noted. For example, rib involvement in one case was better seen on DWI than on CE imaging [Figure 1].

- A 72 year old female with inflammatory left breast cancer. Imaging shows CE versus diffusion weighted imaging in rib involvement. Rib involvement in caption explains (CE) imaging (arrow) (a) is less well appreciated than on diffusion weighted imaging (arrow) (b).
Using the tools available on the Aegis Breast imaging software, regions of interest (ROIs) were manually drawn around regions of qualitative increased signal associated with the mass on relevant diffusion-weighted images minimizing T2 shine-through, and the same ROI was copied to the ADC map. The mean, standard deviation, and range of the ADCs (“restricted diffusion”) in the ROIs were recorded.
Correlations with histopathology and response to NAC were made.
RESULTS
Radiological assessment is summarized in Table 1. Loss of the posterior fat plane could not be determined by DWI alone because of signal attenuation of the anatomy in this region.
MRI acquisition | Imaging feature | Number of cases |
---|---|---|
T2-weighted | Loss of prepectoral fat plane | 23 |
CE MRI | Loss of prepectoral fat plane | 22 |
Enhancement of ribs | 2 | |
Intercostal muscles | 7 | |
Pectoralis muscle | 19 | |
DWI | Loss of prepectoral fat plane | * |
Restricted diffusion in ribs | 2 | |
Intercostal muscles | 6 | |
Pectoralis muscle | 18 |
T2-weighted imaging showed loss of the posterior fat plane in all 23 (100%) cases, and CE imaging showed loss of the posterior fat plane in 22/23 (96%) cases [Figure 2]. In all cases, the identified areas of restricted diffusion correlated with the imaging location of known malignancy. Chest wall involvement (defined as direct invasion of ribs, serratus anterior muscle, or intercostal muscles) was identified in 9/23 (39%) cases by CE imaging and 8/23 (35%) cases by DWI. Pectoralis muscle involvement was identified in 19/23 (83%) cases by CE imaging and 18/23 (78%) cases by DWI. In all cases, the identified areas of restricted diffusion correlated with the imaging location of known malignancy.
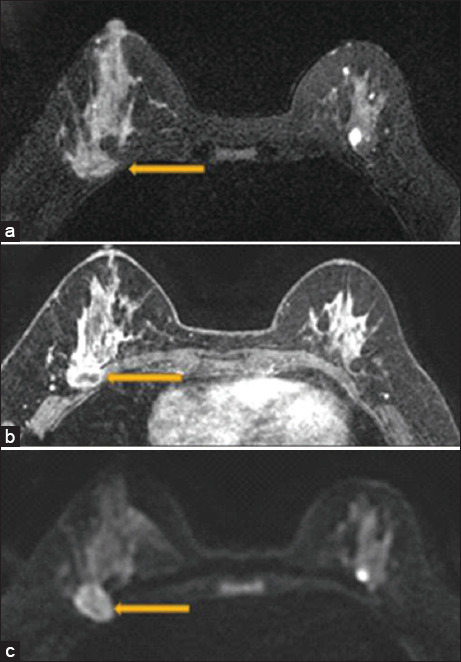
- A 47 year old female with biopsy-proven right breast invasive carcinoma. Breast magnetic resonance imaging showing loss of the posterior fat plane (arrow) on T2 (a), loss of fat plane on postcontrast sequences with pectoralis muscle enhancement (arrow) (b), and diffusion restriction in the pectoralis muscle (arrow) (c). Surgical pathology demonstrated evidence of skeletal muscle involvement.
Apparent diffusion coefficient assessment
Biopsy-proven malignancies were associated with a mean ADC of 1.150 ± 0.42 while radiological chest wall and pectoralis muscle invasions were associated with a mean ADC of 1.285 ± 0.53. The calculated P value of 0.24 supports a null hypothesis that there is no difference between the mean ADC values in the tumor versus the mean ADC values in the chest wall and pectoralis muscle, which is clinically what one would expect.
Pathology
Imaging and surgical pathology were correlated [Table 2]. The 23 tumors included 16 (70%) invasive ductal carcinomas (IDCs), 1 (4%) invasive lobular carcinoma (ILC), 3 (13%) mixed invasive ductal and lobular carcinoma, and 3 (13%) desmoid-type fibromatosis.
Surgical pathology | Number patients | Loss of fat plane on | Involvement of pectoralis muscle and/or chest wall | ||
---|---|---|---|---|---|
T2 images | CE images | Enhancement | Restricted diffusion | ||
Skeletal muscle involvement | 12 | 12 | 12 | 12 | 11 |
No skeletal muscle involvement | 11 | 11 | 10 | 7 | 7 |
Total patients | 23 | 23 | 22 | 19 | 18 |
CE: Contrast-enhanced
Pathology review documented histologic evidence of skeletal muscle involvement in 12 of 23 cases (52%) [Figure 3]. Among these, 12/12 had loss of fat plane on both T2 and CE images, 12/12 were associated with enhancement in the chest wall, and 11/12 had restricted diffusion in the chest wall. Pathologic evidence of skeletal muscle involvement was absent in 11 tumors. However, six of these patients had received NAC prior to surgery which may have influenced the results.
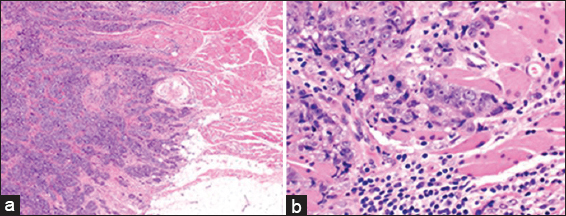
- A 47 year old female with biopsy-proven right breast invasive carcinoma. Low magnification (a: H and E; ×20) and high magnification (b: H and E; ×400) micrographs demonstrate invasive ductal carcinoma, Nottingham grade III (of III) infiltrating mature skeletal muscle.
When assessing for chest wall and pectoralis muscle invasion, DWI had one false-negative exam [Figure 4] compared to seven false-positive examinations [Figure 5]. The sensitivity for contrast enhancement vs. restricted diffusion involving the chest wall or pectoralis muscle was 100% and 92%, respectively. Specificity for both was the same at 36%. The positive predictive value was 63% for contrast enhancement and 61% for restricted diffusion involving the chest wall or pectoralis muscle. The negative predictive value (NPV) was higher, 100% for using contrast enhancement versus 80% for using restricted diffusion to evaluate the chest wall or pectoralis muscle [Table 3].
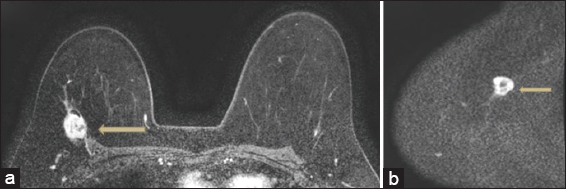
- False negative. 54 year old patient with biopsy proven invasive ductal carcinoma. The patient in this case had loss of fat plane with enhancement in the pectoralis (arrow) noted on postcontrast sequences and tenting of the pectoralis (a). T2 sequences (not shown) also demonstrated loss of fat plane. Based on the consensus read, diffusion weighted imaging was felt to be negative in the chest wall (arrow), likely secondary to poorly defined anatomical landmarks on the diffusion sequences (b). Pathology demonstrated skeletal muscle involvement by tumor.
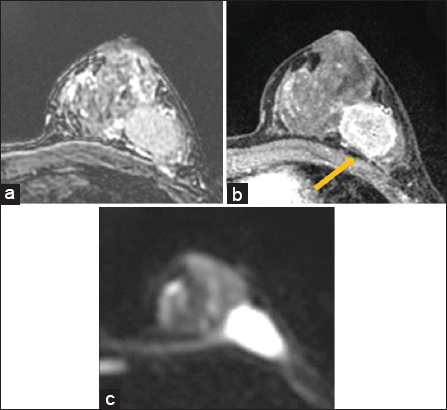
- False positive. 38 year old female with invasive ductal carcinoma of the left breast with metastases to left axillary lymph nodes. Breast magnetic resonance imaging was performed prior to neoadjuvant chemotherapy. There is loss of fat plane on T2 sequences (a), and no enhancement of the pectoralis muscle (arrow) on post contrast sequences (b). Diffusion weighted imaging was interpreted as positive for pectoralis muscle invasion based on consensus read. After neoadjuvant chemotherapy, final surgical pathology did not show evidence of skeletal muscle involvement.
Sensitivity (%) | Specificity (%) | PPV (%) | NPV (%) | |
---|---|---|---|---|
Contrast enhancement | 100 | 36 | 63 | 100 |
Diffusion restriction | 92 | 36 | 61 | 80 |
NPV: Negative predictive value, PPV: Positive predictive value
DISCUSSION
DWI is a well-accepted acquisition in MRI and used extensively in neuroradiology and body MRI, for example in prostate imaging.[3,21-28] In breast MRI, DWI is currently not a required sequence according to the minimum standards set forth by the American College of Radiology. Although breast DWI is not a relatively long scan, the current push for shorter breast MRI examinations to reduce cost and increase accessibility to patients often results in DWI being the first imaging acquisition to omit. As such, abbreviated breast MRIs do not include DWI in its protocol.[29] The utility of DWI in breast MRI largely rests with its correlation to histopathology (specificity) and its added information when intravenous contrast cannot be administered.[8,13,30-33] More recent investigations are looking at diffusion tensor imaging in pregnancy-associated breast cancers when intravenous gadolinium-based contrast agent cannot be administered.[34]
Given that chest wall enhancement which is currently used in imaging to determine chest wall invasion, this study shows that DWI is a useful adjunct series for evaluating far posterior breast tumors on breast MRI as it overall demonstrated comparable sensitivity with CE imaging of the chest wall and pectoralis muscle. The unexpected low specificity (36%) of both CE imaging and DWI was unexpected and is due to the relatively large number of false positives in the calculation, likely secondary to the many patients who received NAC prior to surgery and lack of post NAC MRI. Post NAC MRI was present in only 1/7 patients in whom MRI images suggested pectoralis and chest wall invasion but pathology was negative for skeletal muscle invasion [Figure 6]. What is relatively reassuring are the NPVs of 100% for CE imaging and 80% for DWI in the evaluation of the chest wall or pectoralis muscle. This study corroborates the clinical utility of DWI in diagnostic interpretation of breast MRIs. Used in conjunction with other MRI acquisitions, DWI is perhaps most useful when it is negative, and that seems to be reflected in the NPV in this study.
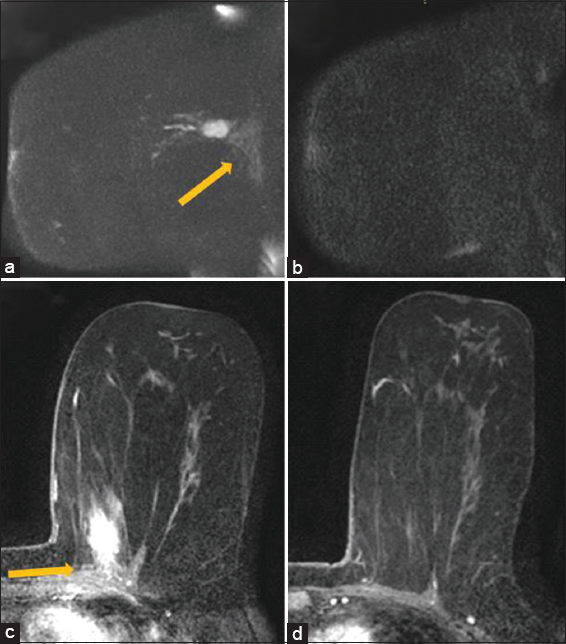
- A 65 year old female with diagnosis of biopsy-proven left breast cancer in the upper inner left breast adjacent to the chest wall. Maximum intensity projection image of a sagittal diffusion weighted imaging sequence (B800 series) demonstrates bright diffusion weighted imaging signal in the lesion with slightly less bright diffusion weighted imaging signal (arrow) in the chest wall (a). This was interpreted as diffusion weighted imaging restriction in the chest wall qualitatively. The apparent diffusion coefficient value in the chest wall was 2.5. Subsequent diffusion weighted imaging image after neoadjuvant chemotherapy demonstrates resolution of previously seen diffusion restriction within the mass and in the chest wall (b). Initial magnetic resonance imaging prior to neoadjuvant chemotherapy demonstrates enhancing mass in the left breast with enhancement extending into the pectoralis muscle (arrow), compatible with chest wall invasion (c). Subsequent magnetic resonance imaging demonstrates resolution of the mass and enhancement in the pectoralis muscle (d). The pathology in this case was negative for skeletal muscle involvement but patient had received neoadjuvant chemotherapy.
Prior studies summarized in Table 4 have established that the ADC values in malignant lesions are lower than in benign lesions.[18,19,33] The ADC values of the 3 benign lesions (desmoids) and 20 malignant lesions (IDC, ILC, and mixed) in this study are similar to prior studies [Table 4] which is reassuring. In known malignancies with chest wall extension, we found no significant difference between the mean ADC values in the tumor versus in the chest wall, which is clinically expected and supports the theory that DWI values are reliable in the identification of chest wall invasion. The information provided by ADC values suggests that it can provide qualitative assurance of favoring malignancy, but a “cut-off” value distinguishing malignant from benign remains difficult to substantiate.
Study | Years | ADC values (×10−3 mm2/s) | B (s/mm2) | ||
---|---|---|---|---|---|
Benign | Malignant | Normal breast tissue | |||
Bogner et al.[18] | 2009 | 1.47±0.21 | 0.99±0.18 | 1.85±0.22 | 50 and 850 |
Pereira et al.[19] | 2009 | 1.44-1.77±0.31-0.44 | 0.68-1.25±0.25-0.28 | 0, 250, 500, 750, and 1000 | |
Guo et al.[15] | 2002 | 1.57±0.23 | 0.97±0.20 | 0, 250, 500, and 1000 | |
Tan et al.[20] | 2014 | 1.49-1.55 | 0.92-1.01 | 500 and 1000 | |
Woodhams et al.[12] | 2005 | 1.12±0.24 | 2.05±0.27 | 0 and 750 | |
Wan et al.[10] | 2016 | 1.27±0.42 | 0.89±0.29 | 0 and 1000 | |
Yoshikawa et al.[8] | 2008 | 1.07±0.19 (IDC); 1.42±0.17 (non-IDC breast cancer) | 1.96±0.21 | 0, 200, 400, 600, and 800 |
ADC: Apparent diffusion coefficient, IDC: Invasive ductal carcinoma
There remains a fairly wide range of b-values used in breast MRI. The authors noted that one of the limitations of DWI is the poor anatomic detail it provides, especially at high B values. This was highlighted in one patient [Figure 5] who demonstrated restricted diffusion in the pectoralis muscle which was not appreciated on DCE imaging. Other useful landmarks in this case such as pectoralis muscle and ribs were difficult to identify in this study. In such cases, T2-weighted, precontrast, and postcontrast images were helpful for correlating DWI findings with anatomy. Additionally, the small sample size over a period of 14 years was confounded by varying DWI acquisition techniques. Pathology assessment of skeletal involvement did not distinguish between pectoralis muscle, serratus anterior muscle, or intercostal muscle involvement, making the determination of strict chest wall invasion ambiguous. This determination was largely made by imaging or if the surgeon provided details of the specimen.
The utility of DWI is important in breast MRI and is best when used in conjunction with T2-weighted imaging and CE imaging, as these sequences aid in depicting anatomic or soft tissue interfaces. This principle is also applicable to MRI of other regions of the body and not limited to breast MRI examinations.
CONCLUSION
Although DWI provides diagnostic information of chest wall invasion almost comparable to CE imaging, DWI remains a largely qualitative assessment that is best read in conjunction with T2-weighted and CE images.
Declaration of patient consent
The authors certify that they have obtained all appropriate patient consent forms. In the form the patient(s) has/have given his/her/their consent for his/her/their images and other clinical information to be reported in the journal. The patients understand that their names and initials will not be published and due efforts will be made to conceal their identity, but anonymity cannot be guaranteed.
Financial support and sponsorship
Nil.
Conflicts of interest
There are no conflicts of interest.
REFERENCES
- Radiologist's role in breast cancer staging:Providing key information for clinicians. Radiographics. 2014;34:330-42.
- [Google Scholar]
- Evaluation of pectoralis major muscle in patients with posterior breast tumors on breast MR images:Early experience. Radiology. 2000;214:67-72.
- [Google Scholar]
- Diffusion weighted magnetic resonance imaging and its recent trend-a survey. Quant Imaging Med Surg. 2015;5:407-22.
- [Google Scholar]
- Diffusion tensor MRI:Preliminary anisotropy measures and mapping of breast tumors. J Magn Reson Imaging. 2010;31:339-47.
- [Google Scholar]
- Quantitative diffusion imaging in breast cancer:A clinical prospective study. J Magn Reson Imaging. 2006;24:319-24.
- [Google Scholar]
- Functional magnetic resonance of human breast tumors:Diffusion and perfusion imaging. Ann N Y Acad Sci. 2002;980:95-115.
- [Google Scholar]
- Unenhanced breast MRI (STIR, T2-weighted TSE, DWIBS):An accurate and alternative strategy for detecting and differentiating breast lesions. Magn Reson Imaging. 2015;33:951-5.
- [Google Scholar]
- Relation between cancer cellularity and apparent diffusion coefficient values using diffusion-weighted magnetic resonance imaging in breast cancer. Radiat Med. 2008;26:222-6.
- [Google Scholar]
- Diffusion-weighted imaging in tissues:Theoretical models. NMR Biomed. 1995;8:289-96.
- [Google Scholar]
- Apparent diffusion coefficient in differentiation between malignant and benign breast masses:Does size matter? Clin Radiol. 2016;71:170-7.
- [Google Scholar]
- Microperfusion-induced elevation of ADC is suppressed after contrast in breast carcinoma. J Magn Reson Imaging. 2009;29:1080-4.
- [Google Scholar]
- Diffusion-weighted imaging of malignant breast tumors:The usefulness of apparent diffusion coefficient (ADC) value and ADC map for the detection of malignant breast tumors and evaluation of cancer extension. J Comput Assist Tomogr. 2005;29:644-9.
- [Google Scholar]
- In vivo diffusion-weighted MRI of the breast:Potential for lesion characterization. J Magn Reson Imaging. 2002;15:693-704.
- [Google Scholar]
- Differentiation between benign and malignant breast lesions using ADC on diffusion-weighted imaging at 3.0 T. Open J Radiol. 2016;6:1-9.
- [Google Scholar]
- Differentiation of clinically benign and malignant breast lesions using diffusion-weighted imaging. J Magn Reson Imaging. 2002;16:172-8.
- [Google Scholar]
- Diffusion-weighted magnetic resonance imaging in focal breast lesions:Analysis of 78 cases with pathological correlation. Radiol Med. 2011;116:264-75.
- [Google Scholar]
- Diffusion-weighted MRI findings of treated and untreated retroperitoneal fibrosis. Diagn Interv Radiol. 2014;20:459-63.
- [Google Scholar]
- Assessment of breast lesions with diffusion-weighted MRI:Comparing the use of different b values. AJR Am J Roentgenol. 2009;193:1030-5.
- [Google Scholar]
- Diffusion-weighted MR for differentiation of breast lesions at 3.0 T:How does selection of diffusion protocols affect diagnosis? Radiology. 2009;253:341-51.
- [Google Scholar]
- Differentiation between benign and malignant breast lesions using quantitative diffusion-weighted sequence on 3 T MRI. Clin Radiol. 2014;69:63-71.
- [Google Scholar]
- Diffusion-weighted imaging in the abdomen and pelvis:Concepts and applications. Radiographics. 2009;29:1797-810.
- [Google Scholar]
- Diffusion weighted imaging of the prostate-principles, application, and advances. Transl Androl Urol. 2017;6:490-8.
- [Google Scholar]
- Clinical utility of diffusion-weighted magnetic resonance imaging in prostate cancer. BJU Int. 2011;108:1716-22.
- [Google Scholar]
- Prostate cancer vs. post-biopsy hemorrhage:Diagnosis with T2-and diffusion-weighted imaging. J Magn Reson Imaging. 2010;31:1387-94.
- [Google Scholar]
- Correlation of diffusion-weighted magnetic resonance data with cellularity in prostate cancer. BJU Int. 2009;103:883-8.
- [Google Scholar]
- Advantages of adding diffusion-weighted magnetic resonance imaging to conventional magnetic resonance imaging for evaluating acute stroke. Arch Neurol. 2000;57:1311-6.
- [Google Scholar]
- Diffusion-weighted MR imaging of intracerebral masses:Comparison with conventional MR imaging and histologic findings. AJNR Am J Neuroradiol. 2001;22:969-76.
- [Google Scholar]
- Abbreviated breast MRI for screening women with dense breast:The EA1141 trial. Br J Radiol. 2018;91:20170441.
- [Google Scholar]
- Advances in breast MRI:Diffusion-weighted imaging of the breast. Breast Cancer. 2008;15:212-7.
- [Google Scholar]
- Detecting breast cancer with non-contrast MR imaging:Combining diffusion-weighted and STIR imaging. Magn Reson Med Sci. 2007;6:21-7.
- [Google Scholar]
- Water diffusion in the different microenvironments of breast cancer. NMR Biomed. 2004;17:170-80.
- [Google Scholar]
- Sensitivity and specificity of unenhanced MR mammography (DWI combined with T2-weighted TSE imaging, ueMRM) for the differentiation of mass lesions. Eur Radiol. 2010;20:1101-10.
- [Google Scholar]
- Noncontrast breast MRI during pregnancy using diffusion tensor imaging:A feasibility study. J Magn Reson Imaging. 2019;49:508-17.
- [Google Scholar]