Translate this page into:
Energy Limits in Second Generation High-pitch Dual Source CT - Comparison in an Upper Abdominal Phantom
Address for correspondence: Dr. Martin Beeres, Department of Diagnostic and Interventional Radiology, Clinic of the Goethe University, Haus 23C UG, Theodor-Stern-Kai 7, Frankfurt - 60590, Germany. E-mail: beeres@gmx.net
-
Received: ,
Accepted: ,
This is an open-access article distributed under the terms of the Creative Commons Attribution License, which permits unrestricted use, distribution, and reproduction in any medium, provided the original author and source are credited.
This article was originally published by Medknow Publications & Media Pvt Ltd and was migrated to Scientific Scholar after the change of Publisher.
Abstract
Objectives:
The aim of our study was to find out how much energy is applicable in second-generation dual source high-pitch computed tomography (CT) in imaging of the abdomen.
Materials and Methods:
We examined an upper abdominal phantom using a Somatom Definition Flash CT-Scanner (Siemens, Forchheim, Germany). The study protocol consisted of a scan-series at 100 kV and 120 kV. In each scan series we started with a pitch of 3.2 and reduced it in steps of 0.2, until a pitch of 1.6 was reached. The current was adjusted to the maximum the scanner could achieve. Energy values, image noise, image quality, and radiation exposure were evaluated.
Results:
For a pitch of 3.2 the maximum applicable current was 142 mAs at 120 kV and in 100 kV the maximum applicable current was 114 mAs. For conventional abdominal imaging, current levels of 200 to 260 mAs are generally used. To achieve similar current levels, we had to decrease the pitch to 1.8 at 100 kV — at this pitch we could perform our imaging at 204 mAs. At a pitch of 2.2 in 120 kV we could apply a current of 206 mAs.
Conclusion:
We conclude our study by stating that if there is a need for a higher current, we have to reduce the pitch. In a high-pitch dual source CT, we always have to remember where our main focus is, so we can adjust the pitch to the energy we need in the area of the body that has to be imaged, to find answers to the clinical question being raised.
Keywords
Abdominal imaging
computer tomography
high-pitch
INTRODUCTION
During the last few years, dual-source high-pitch imaging has become increasingly important in the daily routine of Radiology Departments.[1] In dual-source computed tomography (CT) imaging two ‘sources’ means that two X-ray tubes are in use at an angle of approximately 90 degrees to each other.[1] After introduction of the second generation dual-source CT, about four years ago, increasing pitch (pitch = table mm/n * T mm, where n = No. of slices and T = slice thickness mm) values above the former traditional technical limit of 1.5 in a single-source CT, became possible.[2] When high-pitch imaging is performed, one of the major advantages, compared to conventional single source normal pitch imaging, is the image acquisition time. In high-pitch imaging it is possible to get a significant reduction in motion artifacts.[34567891011121314] This makes high-pitch imaging the technique of choice, especially when regions of the body that are normally subject to motion artifacts have to be examined.[11] Therefore, in clinical practice there are some major regions of interest where high-pitch imaging can demonstrate its advantages, such as, in imaging of the heart, the great thoracic vessels, and the lungs, in patients who have problems holding their breath.[3456789101112131415] As our clinical experience in high-pitched imaging shows — especially in non-electrocardiogram (EKG)-gated high-pitch imaging — the amount of energy that can be applied may be limited by patient habitus.[4] In high-pitch imaging of the lung, these energy factors are not relevant, but in imaging of the upper abdominal tract the maximum applicable energy may cause problems, leading to diagnostic issues. Therefore, the aim of this study was to examine the maximum current levels that can be used in dual-source high-pitch CT during imaging of the upper abdominal tract.
MATERIALS AND METHODS
This study was carried out using an experimental setup that remained constant throughout. An upper-abdominal phantom was placed on the CT table and no alterations were made to this experimental setup during all the CT examinations [Figure 1]. First, the phantom was examined for a scout view. This was followed by a series of high-pitch examinations, beginning with a pitch of 3.2 [Table 1]. This value was reduced in steps of 0.2, with each successive test, ending at a pitch of 1.6 in the final examination [Tables 2 and 3]. All investigations were carried out in the same scan range, once with a tube voltage of 120 kV, and once with 100 kV (Modes 1 and 2, Table 1). The tube charge in mAs (= mA multiplied by the rotation time) was always adjusted to the maximum that the CT machine could perform at, without using the dose modulation software (Care Dose 4D), so that the exact tube maximum could be reached. To achieve the maximum current, the mAs value was increased stepwise until a malfunction message appeared on the screen. One step downward from the level producing an error-message was chosen for the examinations, and this was taken as the maximum current that the CT machine was able to apply.
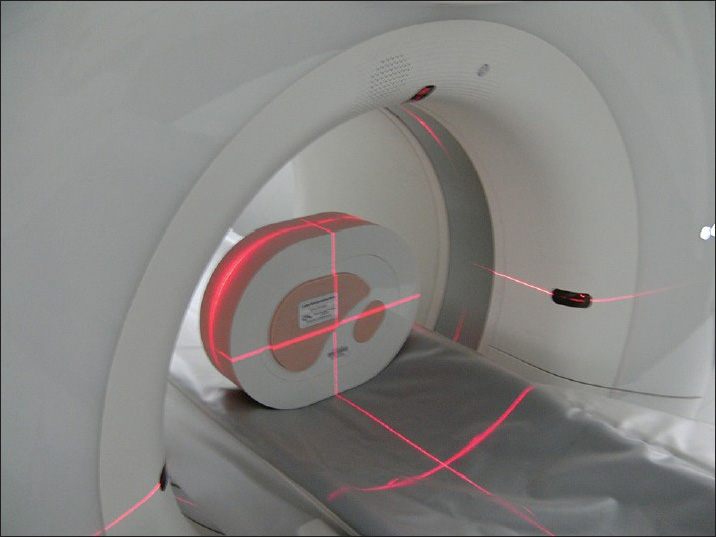
- Adjustment of the phantom.

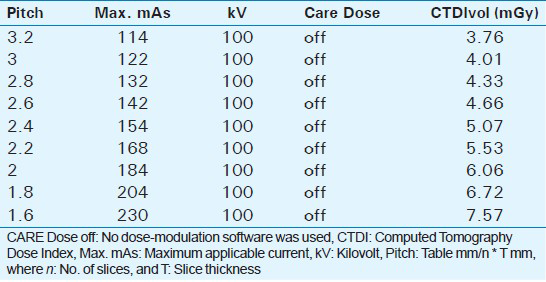
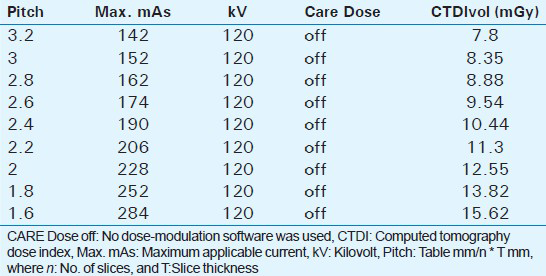
The images obtained were reconstructed in a conventional filtered back-projection recon kernel B30, as frequently used for imaging soft tissue. The images were assessed in slices/increments of 5 mm/5 mm as transversal cross-sectional images. For further evaluation coronal and sagittal images in slices/increments of 2 mm/2 mm were reconstructed in the same manner.
Measurements
As objective measures of image quality of the examination series, several region-of-interest (ROI) measurements, using the circle tool and a Picture Archiving and Communication System (PACS) workstation (Centricity 4.2, General Electric Healthcare, Dornstadt, Germany), were performed by a radiologist, who had four years of experience in CT. The mean attenuation values and standard deviation were recorded and displayed in Hounsfield Units (HU). The background noise (BN) was determined as the standard deviation of air measured in front of the phantom. The attenuation at different locations in the phantom were measured (liver parenchyma, liver metastases (hyper- and hypo-dense examples), muscle tissue, cutaneous fat tissue, cutaneous metastases (hyper- and hypodense examples), as also the air in front of the phantom). The ROI measurements were drawn as large as possible, to include as much of the corresponding area of interest as feasible. To minimize bias from single measurements, we calculated the average of four measurements for each ROI. On the basis of these measurements, the signal-to-noise ratio (SNR) was determined according to the following equation: SNR = Attenuation/BN. To calculate the contrast-to-noise ratio (CNR)- value, we measured the attenuation and the HU and SD of attenuation of the surrounding muscle tissue (ROI muscle) compared with the attenuation of the hyperdense subcutaneous lesions [Figures 2 and 3]. CNR was calculated as CNR = ((ROI subclesion-ROI muscle)/image noise).
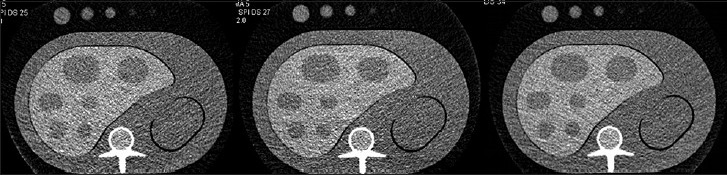
- Images at different pitch values (100 kV, A = Pitch 3.2; B = Pitch 2.8, C = Pitch 2.2; B30 Kernel).
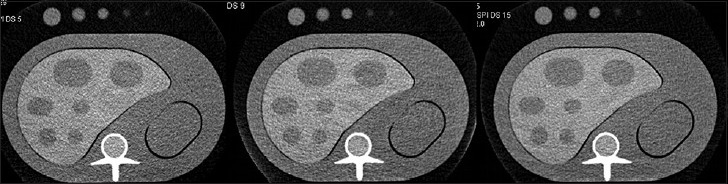
- Images at different pitch values (120 kV, A = Pitch 3.2; B = Pitch 2.8, C = Pitch 2.2).
Subjective image quality rating was conducted in a blind fashion by two independent radiologists, with three and four years of experience, respectively, in general CT imaging. The rating was done according to a five-point scale as follows: 1 = excellent/no artifacts, 2 = good/hardly any artifacts, 3 = moderate/few artifacts, 4 = fair/many artifacts, 5 = unacceptable.
Statistical analysis
Categorical variables were reported as counts. Continuous variables were expressed as median and range. Exemplary: Attenuation values, SNR, CNR, subjective image quality score, DLP (Dose Length Product) and CTDIvol (Computed Tomography Dose Index). Analyses were performed using a dedicated software (BiAS 9.14, Epsilon Verlag, Germany). For statistical comparison a Kruskal-Wallis Test was performed. A Cohen's kappa analysis was performed to determine the interobserver agreement for subjective image quality scoring.
RESULTS
It became apparent that the maximum applicable energy, especially at increasing pitch levels, was limited. At a pitch factor of 3.2 it was possible to apply a maximum of 142 mAs at 120 kV and 114 mAs at 100 kV. All measurements were performed without the use of a dose modulating software (Care Dose 4D, Siemens, Forchheim, Germany) to obtain a value for the maximum energy that the CT machine was able to deliver. As the applicable energy decreased, while increasing the pitch factor, the image noise increased. The SNR and CNR values decreased, while the pitch factors increased [Figures 4 and 5]. When discussing the increasing imaging noise, we should consider the reduction in image quality. As expected, in the subjective image analysis, we saw a near linear correlation between the increase in pitch factor and a corresponding decrease in the applicable energy, to decreasing image quality). The linear correlation did display a dip in the curve, and this dip occurred at a pitch factor of 2.8 [Figure 6]. Therefore, performing examinations at a pitch factor of 2.8 resulted in a better image quality compared to the pitch values nearby. Thus, this remained an interesting point for future investigations, although we could not immediately envisage why at that particular pitch value the image quality was rated to be better compared to the other values. Therefore, there might be an advantage in conducting a study exploiting a more overlapping dataset. To reduce the image noise seen in high-pitch imaging, it had already been proved that iterative reconstruction algorithms had positive effects on image quality.[1617] In our study, we reconstructed the images in the B30 kernel (medium-smooth kernel), which were often used for abdominal imaging.[18] With regard to the image noise, we calculated the SNR, CNR, and additionally evaluated the image quality in grades from one to five, as explained above [Figures 4–6]. The interobserver agreement was good, with a value of Cohen's kappa of 0.8.
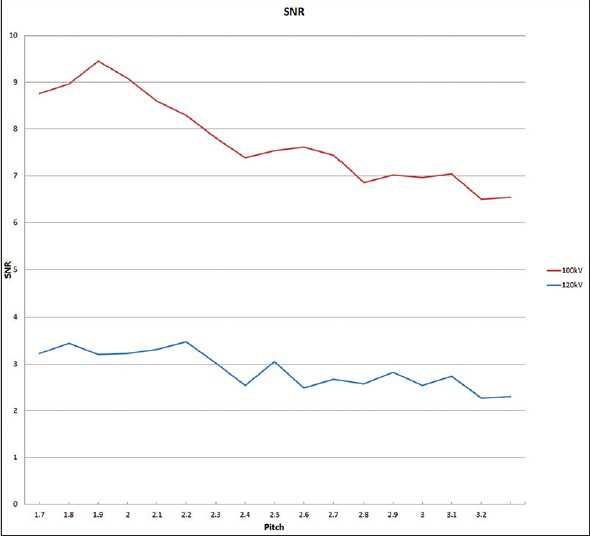
- SNR-Values between 100 and 120 kV examinations.
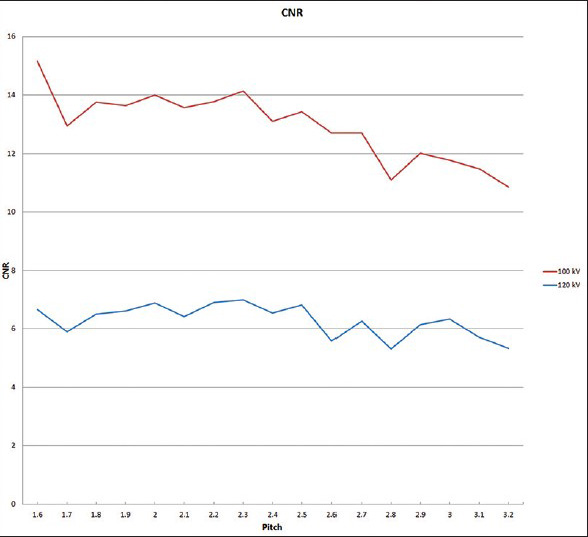
- CNR-Values between 100 and 120 kV examinations.
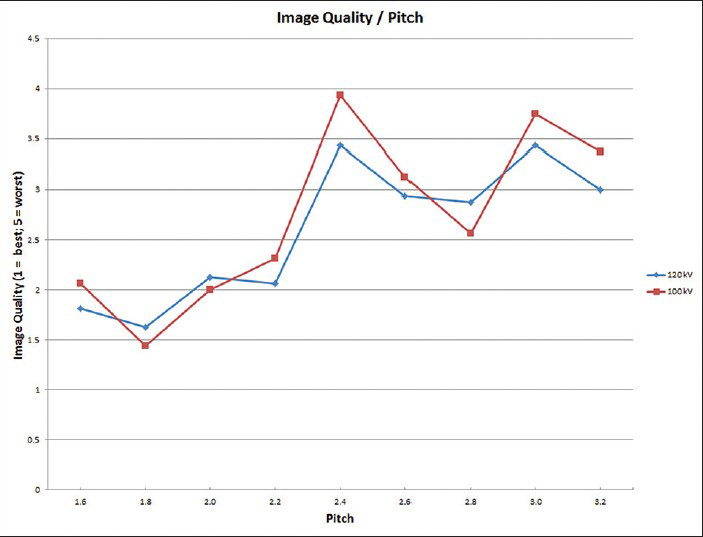
- Subjective image quality at different pitch factors (Quality Rating Scale from 1 to 5 = Y-axis: 1 = excellent image quality, 5 = worst image quality). The red line represents the 100 kV group, the blue line represents the 120 kV group.
DISCUSSION
The main result of our study was that the energy in dual source high-pitch mode was definitely limited. As shown in Tables 2 and 3, the maximum applicable current that could be supplied by the machine, especially beyond a pitch of 3.0, had to be kept in mind when imaging at those pitch values. The scanner studied here was often working at its absolute limits; these limits were a result of the accelerated rotation time combined with fast table movement.[19] Together, the time during which radiation was applied to the patient, and consequently the image acquisition time during which the detector was absorbing the X-rays, remained so brief that the tubes were often working at a current above 1000 mA. These values were close to the total energy limits of the tube. Therefore, when we tried to go beyond these limits, the scanner produced a malfunction message. Thus, this represented one major cause for the dose savings described in the recent literature.[1220] In a conventional single-source mode, a CT machine was able to deliver as much energy as was needed, and so gained a constant noise-level, and this could be adjusted using the Dose-Modulation software (such as Care Dose 4D).[21] In high-pitch mode, the machine, at some point during examination of the patient, was not able to deliver the energy that was necessary and so a malfunction message was displayed, and as a result the examination had to be performed with ‘under radiation’ in some areas of the body, resulting in less total radiation exposure.
In our examination, we wanted to rule out the limits of the scanner, so we performed all our scans without the use of the dose-modulating software. The use of this software would have meant that we would have been unable to produce valid results and it would not have been possible to draw conclusions that would have been relevant to clinical practice from our results. This was on account of various patient features, especially the body mass index, as this was the major influential factor of the dose-modulation software, which adjusted the energy levels to keep the noise levels stable.[21] When the dose-modulating software is used, the tube or the scanner can be adjusted to higher mAs values, as we observed, and this is in agreement with the recent publications in the field of high-pitch imaging.[812] However, even in the field of high-pitch imaging, when using the dose-modulating software, the energy is still limited. Naturally, determining these values by using the dose-modulation software is more complicated, because other influential factors have to be kept in mind (e.g., patient size, weight, and the region that will be examined). Overall, for the evaluation of the maximum applicable energy when using dose modulating software, further studies have to be performed, but in our view the study here may represent a first step.
With respect to the image quality rating, looking at Figure 6, there is a dip in the curve at pitch values between 2.6 and 2.8. We cannot explain why at exactly these pitch values the image quality is rated better compared to the other values. Thus, it may be advantageous to conduct a study utilizing a more overlapping dataset. Performing examinations at a pitch factor between 2.6 and 2.8 resulted in an image quality nearly equal to a pitch factor of 2.2.
In recent times, many studies concerning high-pitched imaging have been published. Most of them focus on imaging of the heart and the vessels of the thorax.[34567891011121314] In some studies, the limitation of energy is described and the scanner is adjusted to values that the machine is able to perform, however, a controlled study for the maximum applicable energy has not yet been published. In our study we wanted to determine the limits of high-pitch imaging and the consequences for image quality.
As the ongoing progress in a multi-slice CT enables us to use a more sophisticated CT scanner technology, one of the recent technologies uses wide detector arrays, up to 16 cm, to cover a wide area of the body with one rotation.[22] However, this is a different approach when compared to a dual-source CT, because this remains a one-tube detector system, whereas, in a dual-source CT there are two tube-detector systems in use, at about 90-degree angles to each other.[1] Comparisons between both techniques may be interesting for further research projects.
Limitations
One limitation of our study was that we did not compare the use of the dose-modulating software with the dose modulating software mode when it was not used. However, our main goal was to show how much energy could be applied in a stable setting, and therefore, we performed our examinations without the dose modulation software. Of course, the energy levels when using the dose modulating software would be limited too.
Another limitation of the CT scanner is the width of the second detector, which only covers 33 cm. Another limitation we show in our study is the maximum energy that can be applied to the patient. In a conventional single source mode, the maximum applicable energy is not generally a concern, because the scanner, depending on the rotation time, is normally able to deliver as much energy as is needed to examine the patient, irrespective of their height and weight. In high-pitch imaging this is not always possible.
CONCLUSION
High-pitch imaging, in departments where a CT machine that is able to work in this mode is available, is already a standard examination tool for selected clinical indications. As the high-pitch mode is used more routinely, it becomes increasingly important to examine the limitations of high-pitch imaging. Therefore, in some cases, high-pitch imaging is a good solution for imaging patients, but in other cases, especially in abdominal imaging, it may be desirable to apply more energy than the CT is able to deliver. Altogether, not every clinical question can be answered in a way that it could be in conventional single source mode CT. The limited energy, as well as the detector width, have to be kept in mind when examining at high-pitch.
Available FREE in open access from: http://www.clinicalimagingscience.org/text.asp?2015/5/1/2/150441
Source of Support: Nil
Conflict of Interest: None declared.
REFERENCES
- Dual-source spiral CT with pitch up to 3.2 and 75 ms temporal resolution: Image reconstruction and assessment of image quality. Med Phys. 2009;36:5641-53.
- [Google Scholar]
- Diagnostic image quality of a comprehensive high-pitch dual-spiral cardiothoracic CT protocol in patients with undifferentiated acute chest pain. Eur J Radiol. 2010;81:3697-702.
- [Google Scholar]
- High-pitch dual-source CT angiography of the whole aorta without ECG synchronisation: Initial experience. Eur Radiol. 2012;22:129-37.
- [Google Scholar]
- Thoracic aorta: Prospective electrocardiographically triggered CT angiography with dual-source CT-feasibility, image quality, and dose reduction. Radiology. 2010;255:207-17.
- [Google Scholar]
- High-pitch dual-source CT angiography of the thoracic and abdominal aorta: Is simultaneous coronary artery assessment possible? AJR Am J Roentgenol. 2010;194:938-44.
- [Google Scholar]
- High-pitch dual-source CT coronary angiography: Systolic data acquisition at high heart rates. Eur Radiol. 2010;20:2565-71.
- [Google Scholar]
- High-pitch dual-source CT angiography of the aortic valve-aortic root complex without ECG-synchronization. Eur Radiol. 2011;21:205-12.
- [Google Scholar]
- Prospectively ECG-triggered high-pitch spiral acquisition for coronary CT angiography using dual source CT: Technique and initial experience. Eur Radiol. 2009;19:2576-83.
- [Google Scholar]
- High-pitch spiral computed tomography: Effect on image quality and radiation dose in pediatric chest computed tomography. Invest Radiol. 2011;46:116-23.
- [Google Scholar]
- High-pitch thoracic CT with simultaneous assessment of coronary arteries: Effect of heart rate and heart rate variability on image quality and diagnostic accuracy. JACC Cardiovasc Imaging. 2011;4:602-9.
- [Google Scholar]
- Saving dose in triple-rule-out computed tomography examination using a high-pitch dual spiral technique. Invest Radiol. 2010;45:64-71.
- [Google Scholar]
- Coronary artery stent imaging with 128-slice dual-source CT using high-pitch spiral acquisition in a cardiac phantom: Comparison with the sequential and low-pitch spiral mode. Eur Radiol. 2010;20:2084-91.
- [Google Scholar]
- Dual source multidetector CT-angiography before Transcatheter Aortic Valve Implantation (TAVI) using a high-pitch spiral acquisition mode. Eur Radiol. 2012;22:51-8.
- [Google Scholar]
- Intravenous contrast material administration at high-pitch dual-source CT pulmonary angiography: Test bolus versus bolus-tracking technique. Eur J Radiol. 2012;81:2887-91.
- [Google Scholar]
- Evaluation of two iterative techniques for reducing metal artifacts in computed tomography. Radiology. 2011;259:894-902.
- [Google Scholar]
- Multi-slice spiral CT of the abdomen in oncological patients: Influence of table support and detector configuration on image quality and radiation exposure. Rofo. 2001;173:373-8.
- [Google Scholar]
- Pushing the envelope: New computed tomography techniques for cardiothoracic imaging. J Thorac Imaging. 2010;25:100-11.
- [Google Scholar]
- Accuracy, image quality, and radiation dose of prospectively ECG-triggered high-pitch dual-source CT angiography in infants and children with complex coarctation of the aorta. Acad Radiol. 2014;21:1248-54.
- [Google Scholar]
- Dose reduction in CT examination of children by an attenuation-based on-line modulation of tube current (CARE Dose) Eur Radiol. 2002;12:1571-6.
- [Google Scholar]