Translate this page into:
Factors Affecting Radiation Dose in Computed Tomography Angiograms for Pulmonary Embolism: A Retrospective Cohort Study
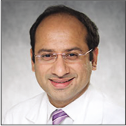
*Corresponding author: Prashant Nagpal, Department of Radiology, University of Iowa Hospitals and Clinics, Iowa City, United States. drprashantnagpal@gmail.com
-
Received: ,
Accepted: ,
How to cite this article: Nagpal P, Priya S, Eskandari A, Mullan A, Aggarwal T, Narayanasamy S, et al. Factors Affecting Radiation Dose in Computed Tomography Angiograms for Pulmonary Embolism: A Retrospective Cohort Study. J Clin Imaging Sci 2020;10:74.
Abstract
Objectives:
Computed tomography pulmonary angiogram (CTPA) is one of the most commonly ordered and frequently overused tests. The purpose of this study was to evaluate the mean radiation dose to patients getting CTPA and to identify factors that are associated with higher dose.
Material and Methods:
This institutionally approved retrospective study included all patients who had a CTPA to rule out acute pulmonary embolism between 2016 and 2018 in a tertiary care center. Patient data (age, sex, body mass index [BMI], and patient location), CT scanner type, image reconstruction methodology, and radiation dose parameters (dose-length product [DLP]) were recorded. Effective dose estimates were obtained by multiplying DLP by conversion coefficient (0.014 mSv•mGy−1•cm−1). Multivariate logistic regression analysis was performed to determine the factors affecting the radiation dose.
Results:
There were 2342 patients (1099 men and 1243 women) with a mean age of 58.1 years (range 0.2–104.4 years) and BMI of 31.3 kg/m2 (range 12–91.5 kg/m2). The mean effective radiation dose was 5.512 mSv (median – 4.27 mSv; range 0.1–43.0 mSv). Patient factors, including BMI >25 kg/m2, male sex, age >18 years, and intensive care unit (ICU) location, were associated with significantly higher dose (P < 0.05). CT scanning using third generation dual-source scanner with model-based iterative reconstruction (IR) had significantly lower dose (mean: 4.90 mSv) versus single-source (64-slice) scanner with filtered back projection (mean: 9.29 mSv, P < 0.001).
Conclusion:
Patients with high BMI and ICU referrals are associated with high CT radiation dose. They are most likely to benefit by scanning on newer generation scanner using advance model-based IR techniques.
Keywords
Radiation
Computed tomography angiography
Pulmonary embolism
Diagnostic imaging
Radiobiology
INTRODUCTION
Chest pain is the second most common cause of emergency room visits by patients amounting for nearly 6 million visits annually.[1] Acute pulmonary embolism (PE) is frequently in the differential diagnosis in patients with chest pain. Computed tomography pulmonary angiography (CTPA) is the test of choice to rule out acute PE in these patients. It has been shown that the use of CTPA has significantly increased over time.[2-5] Studies have shown a true positive rate of less than 2% for diagnosis of acute PE in patients getting CTPA examination[6,7] These studies highlight overutilization of imaging, and a lack of adherence to the use of clinical decision support for ordering CTPA exams. Interestingly, the increase in utilization of CTPA has not shown to increase the diagnostic yield.[8] Due to non-adherence to clinical decision support, there is increased likelihood of detection of low risk PE which are not associated with the right heart strain or myocardial injury.[9]
CT scanners utilize ionizing radiation which has a potential to cause cancers.[10] The radiation induced risk to an individual patient is difficult to measure, but a multicenter study estimated that one in 330 females undergoing CTPA at the age of 20 years will potentially develop radiation-induced cancer.[11] CTPA radiation dose varies among different institutions due to differences in the imaging protocol, such as tube current and voltage parameters.[12] Hence, a precise estimate of cancer-risk from CTPA studies is difficult to measure and attribute.
There have been prior phantom[13] and patient studies[14-17] evaluating the differences in radiation doses between the image reconstruction techniques (filtered back projections [FBP] vs. iterative reconstruction [IR]), but the studies comparing differences in radiation doses based on scanner detectors combined with types of image reconstruction as well as patient characteristics on such scanners (which is close to the real world clinical imaging practice) are lacking. Understanding how patient characteristics and CT scanner factors affect the radiation dose is a vital first step toward dose reduction. The purpose of this study was to measure the patient radiation dose from CTPA exams and to determine the effect of the type of scanner (number of detectors, sources, and the image reconstruction algorithms) and various patient characteristics on radiation dose.
MATERIAL AND METHODS
Data collection
This is retrospective single center cohort study approved by the local Institutional Review Board (IRB). The requirement for an informed consent was waived by the IRB. Radiology and hospital information database was utilized to identify patients that underwent CTPA between 2016 and 2018 meeting the inclusion/exclusion criteria. Inclusion criterion was patients getting CTPA exam to rule out PE. Any double rule out studies (rule out PE and acute aortic syndrome), non-diagnostic exams, or To analyze patient-related factors that would affect the radiation dose, patient age, patient location (outpatient, emergency department, intensive care units [ICU], and inpatients [IN] other than ICU [IN]), gender, and body mass index (BMI) were recorded.
Attenuation-based tube current modulation and tube potential selection (CARE Dose 4D, CARE kV, Siemens Healthcare) are standard in our PE evaluation CTPA protocol, irrespective of the patient or the scanner model. The dose-length product (DLP) for each patient in the study was recorded from the CT scanner and the effective dose (mSv) was calculated based on established k-conversion factor for the chest using the following formula: DLP (mGy•cm) × 0.014 mSv/mGy•cm.[18] Effective dose estimation (rather than DLP) allows comparison with other imaging modalities. The CTPA protocol at our institution is summarized in Table 1.
Scan range | Lung apices to diaphragm |
---|---|
Scan type | Arterial phase (bolus triggered) |
kVp | Care kV (reference kV 120) |
Effective mAs | CareDose (reference mA 200) |
Collimation | Scanner dependent |
Pitch value | 1.0 (Dual source scanners) 0.8 (Single source scanners) |
Trigger | Main pulmonary artery at 200 HU |
Contrast type | Iopamidol 370 mg/mL (Bracco Diagnostic, Princeton, New Jersey, USA) |
Injection rate | 4.0–5.0 mL/s |
Contrast injection | Weight based contrast loading <60 kg: 1 mL/kg >60 kg: 100 mL |
Respiratory phase | Patient instructed to stop breathing |
Scan direction | Craniocaudal |
To analyze the effect of the type of CT scanner (and its image reconstruction algorithm), information about the CT scanner on which the CTPA exam was performed was also recorded. The exams were performed with four types of CT scanners – 64-slice single source scanner with FBP (64SSwFBP, Siemens Somatom Definition AS, Siemens Healthcare), 64-slice single source scanner with Sinogram Affirmed IR (64SSwSAFIRE, Siemens Somatom Definition AS, Siemens Healthcare), 128-slice single source with Sinogram-Affirmed IR n (128SSwSAFIRE, Siemens Definition AS+, Siemens Healthcare), and third generation dual source scanner with model-based Advanced Modeled IR (DSwADMIRE, Siemens Somatom Force, Siemens Healthcare).
Data analysis
The overall effective radiation dose (in mSv) for CTPA examinations was assessed for the entire group. The mean, median, range, and standard deviation were calculated. The radiation dose was determined for various age (<20 year to >90 years and interval every 10 year) and BMI intervals (<15 to > 60 Kg/m2 and interval every 5). For appropriate comparison, the relationship of dose with age-groups (divided into pediatric [<18 years], young adults [18–35 years], middle-age, and elderly adults [>35 years]) and BMI groups (normal weight [<25 kg/m2], overweight and class I and II obesity [25–40 kg/m2], and morbidly obese [>40 kg/m2]) was measured.
Statistical analysis
Statistical analysis reported in our study was performed by biostatistician (A.M) using R software (version–3.5.0, R Foundation for Statistical Computing, Vienna, Austria). Normality was tested using box plots. Continuous variables were expressed as means ± standard deviations or medians and interquartile ranges, depending on distribution. Categorical data were summarized as number and percentage. A multivariate logistic regression analysis was performed to determine what factors affect the radiation dose for CTPA examination. As the distribution of effective dose was right skewed, a linear model was fit to the log-transform of effective dose. All post hoc pairwise comparisons were then performed using Tukey’s honestly significant difference with a false discovery rate correction to compensate for the large number of factor levels being compared. Statistical significance was assumed for P < 0.05.
RESULTS
Patient characteristics
A total of 2342 patients were included in the study. There were 1243 (53.1%) females and 1092 (46.9%) males. The mean age of the population was 58.1 years (SD 17.3, range 0–104 years). Mean BMI was 31.3 (SD 9.9, range 12–91.5). Most patients were over 35-year age (2051, 87.6%) and BMI between 25 and 40 (1309, 55.9%). Table 2 summarizes the patient characteristics.
Total patients | 2342 |
---|---|
Gender | |
Females | 1243 (53.1) |
Males | 1099 (46.9) |
Age (mean ± SD) | 58.1±17.3 |
Age range (years) | 0–104 |
Age groups under 18 | 21 |
18–35 | 270 |
>35 | 2051 |
BMI (mean±SD) | 31.3±9.9 |
<25 | 651 |
25–40 | 1309 |
>40 | 382 |
Scanner types with exams performed | |
64SSwFBP | 59 |
64SSwSAFIRE | 109 |
128SSwSAFIRE | 1001 |
DSwADMIRE | 1173 |
Patient locations | |
Emergency department | 1352 |
Outpatients | 189 |
Inpatients other than ICU | 533 |
Intensive care unit | 268 |
Mean radiation dose
The mean DLP was 394 mGy•cm (median 305, range 7–3072). The effective radiation dose (DLP [mGy•cm] × 0.014 mSv/mGy•cm) was 5.512 mSv (median dose 4.27 mSv; dose range 0.1–43.0 mSv).
Patient factors affecting radiation dose
The mean effective radiation dose for the under 18 age group was 3.18 mSv, which was significantly lower than the average dose for the 18–35 groups (mean: 5.24 mSv, P < 0.001) and over 35 groups (mean: 5.57 mSv, P < 0.001). There was no significant difference between the 18 and 35 groups and over 35 groups [Figure 1]. Multivariate analysis shows that on average, patients <18 years received 84.2% less radiation than patients between 18 and 35 years and 86.6% less radiation as compared to >35-year-old patients.
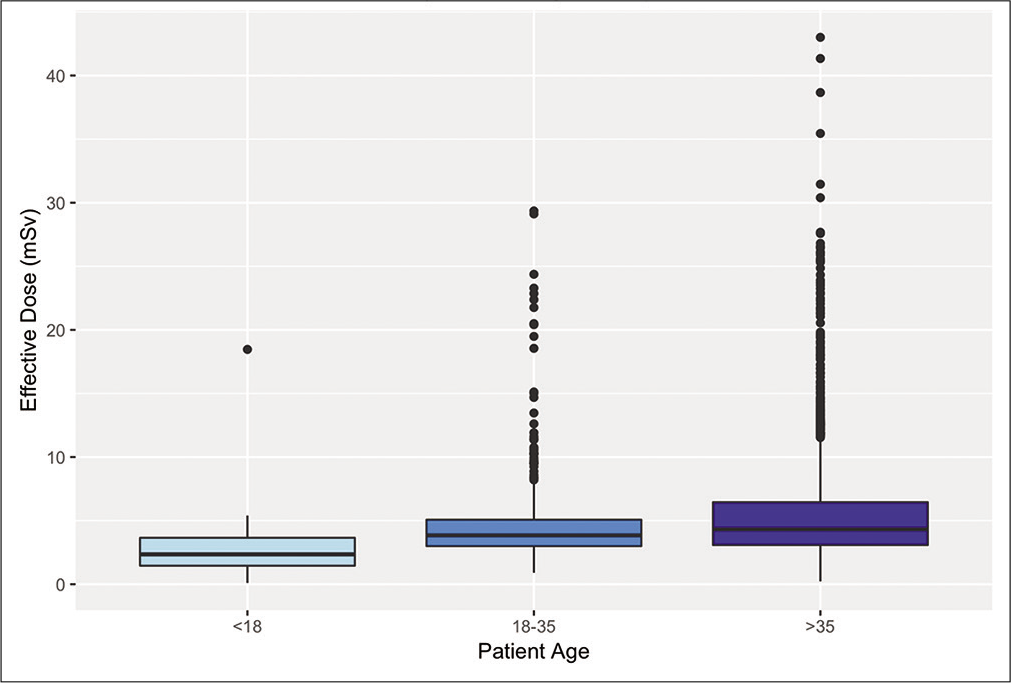
- Box-plot diagram showing effective dose administered among different age-groups (<18 years, 18–35 years, >35 years). Patients <18 years received significantly less radiation dose (P < 0.05) as compared to 18–35 group and over 35 groups. No significant difference between the 18 and 35 groups and over 35 groups.
The mean effective radiation dose to male patients was 5.91 mSv. This was found to be significantly higher than the average dose to female patients (mean: 5.18, P < 0.001). The multivariate analysis showed that males received 25.5% more radiation on average than females.
Patient’s body habitus was a strong predictor for radiation dose with a mean dose of 10.25 mSv for morbidly obese patients (BMI > 40 kg/m2) which was significantly higher than radiation dose for overweight and obesity Classes I and II (BMI 25–40 kg/m2, mean: 5.28 mSv, P < 0.001) as well as patients with BMI less than 25 kg/m2 (mean 3.21 mSv, P < 0.001) [Figure 2]. The linear regression model showed that change of BMI from a normal weight (BMI<25 kg/m2) to overweight or Classes I and II obesity (BMI 25–40 kg/m2) was associated with an increase in radiation dose with CTPA exam by 67.5%. Similarly, a change in body habitus from overweight and Classes I and II obesity (BMI 25–40 kg/m2) to morbid obesity (BMI >40 kg/m2) was associated with a further 89.6% increase in radiation.
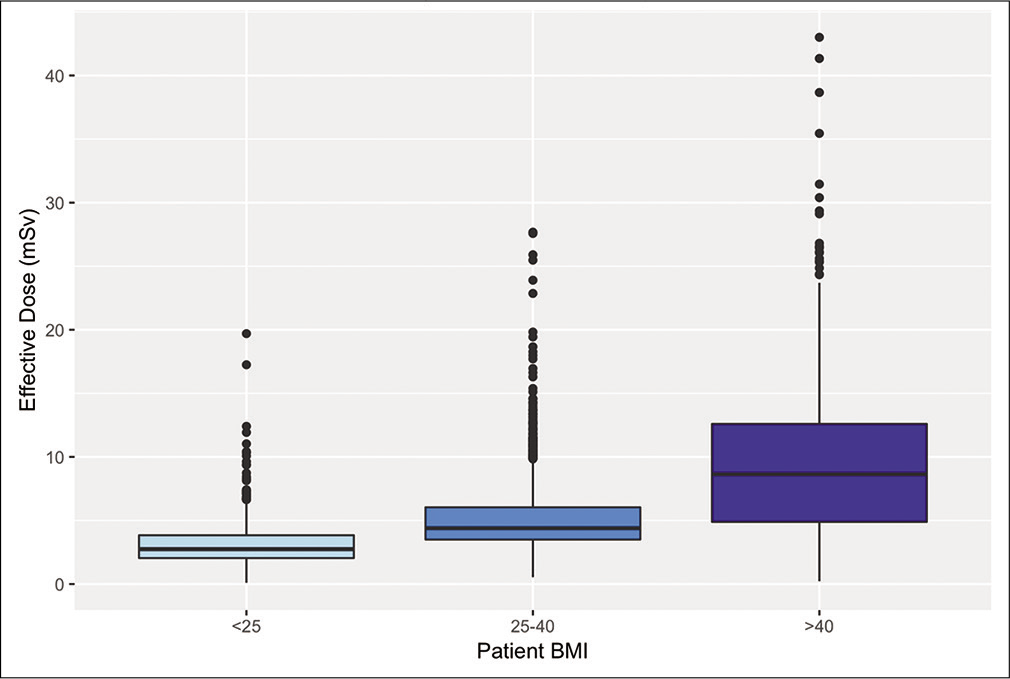
- Box-plot diagram showing effective dose administered among different body mass index (BMI) groups (<25, 25–40, and >40 kg/m2). Patients with BMI >40 kg/m2 received significantly higher radiation dose (P<0.001) as compared to 25–40 kg/m2 group and <25 kg/m2 group.
For the location at which the patient was admitted, ICU patients were associated with the highest radiation dose at an average of 6.16 mSv, compared to an average of 5.43 mSv for all other locations (P < 0.001) [Figure 3]. ICU patients were associated with an 11.1% increase in radiation dosage, according to the multivariate regression analysis.
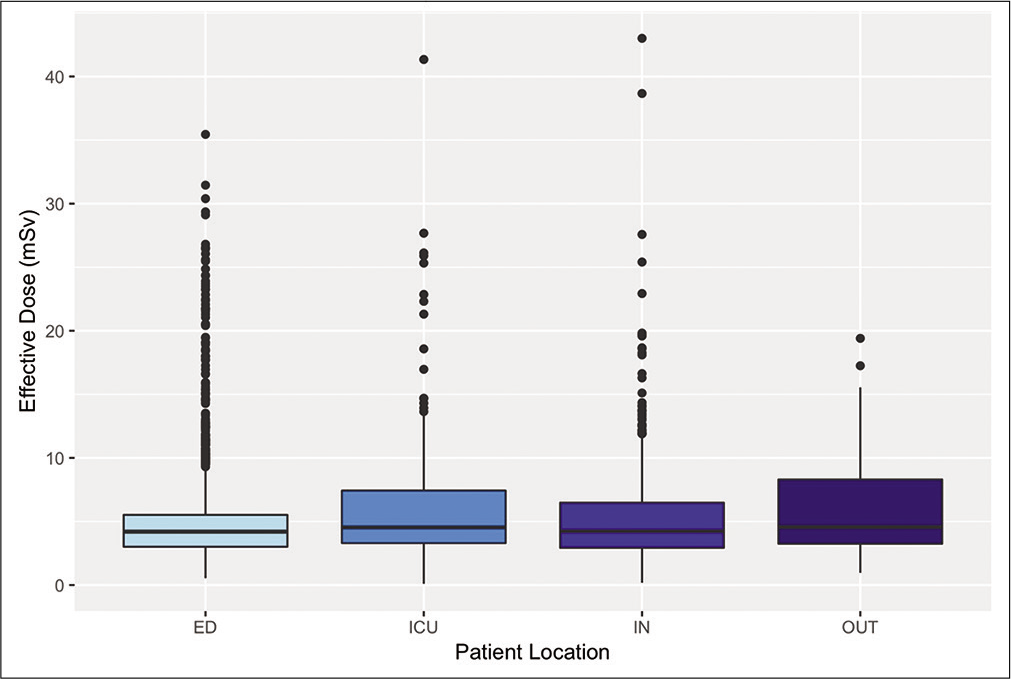
- Box-plot diagram showing effective dose administered among different patient locations. Patients referred from intensive care unit received significantly higher dose (P < 0.001) as compared to an average of all other locations.
CT scanner influence on radiation dose
Among the type of scanners used, the DSwADMIRE scanner had an average effective dose of 4.90 mSv, which was significantly lower than the 128SSwSAFIRE scanner (mean: 5.94 mSv, P < 0.001), 64SSwSAFIRE scanner (mean: 6.08 mSv, P < 0.001), and 64SSwFBP scanner (mean: 9.29 mSv, P < 0.001). The 64SSwFBP scanner was associated with a significantly higher amount of radiation than all other scanners (P < 0.001 for all). There was no significant difference in the radiation dose between the 64SSwSAFIRE and 128SSwSAFIRE scanners [Figure 4]. The linear regression model showed that if CTPA exam was performed on a DSwADMIRE scanner (keeping everything else same), the radiation dose decreased by 18.4% as compared to 128SSwSAFIRE and 54.3% as compared to 64SSwFBP.

- Box-plot diagram showing effective dose administered among different scanner and image reconstruction types. 64-slice scanners with filtered back projection (64SSwFBP) were associated with highest radiation dose (P < 0.001) and dual source scanner with model-based iterative reconstruction (DSwADMIRE) was associated with the lowest radiation dose. No significant dose difference was seen between 64-slice and 128-slice single source scanners with statistical iterative reconstruction algorithm (64SSwSAFIRE, 128SSwSAFIRE).
DISCUSSION
Our study shows that even with a uniform single institution CTPA protocol with automated CT tube current and tube potential modulation – patient characteristics, CT scanner generation, and image reconstruction techniques significantly influence radiation dose to the patients. CTPA is often debated to be an over utilized test with a few recent studies showing a true positive rate of less than 2%.[4,6,7] In addition, there is a wide variation (1.3–10.9%) in true positive CTPA rates between various centers within the US, highlighting practice and imaging utilization differences among different sites.[5,19,20] Like with any other imaging technique, judicious and appropriate use of CTPA is essential, as it has potential side effects that are very difficult to measure and may have a very long latency. The reason for CTPA overutilization is multifactorial and may be difficult to overcome in the US largely due to practice of defensive medicine.[4,21] There are two important patient detriments from overuse of CTPA – radiation induced cancer[11,22] and contrast-induced nephropathy (CIN).[23] While CIN was estimated to be between 13 and 24% among patients getting CT for PE evaluation,[23,24] the recent studies have shown that this was an overestimate and the true contrast-induced acute kidney injury is very difficult to measure and is rare.[25] As per a recent multi-society consensus statement,[25] the true risk of contrast-induced acute kidney injury is nearly 0% at estimated glomerular filtration rate (eGFR) greater than or equal to 45, 0–2% at eGFR 30–44, and 0–17% at eGFR less than 30 mL/min/1.73 m2.
Attempts have been made to quantify diagnostic radiation-induced cancers in the past, but no uniform direct causal measure has been established due to individual variations in dose and radio sensitivity. In a multi-center study, it was estimated that one in 330 females undergoing CTPA at the age of 20 years potentially develops radiation-induced cancer.[11] Another single center study estimated that lifetime attributable risk of cancer mortality is 57/100,000 for females 17–19 years old and 8/100,000 for males and females 80–89 years.[22] Despite these potentially harmful effects of CTPA exam, benefit-to-risk models have shown that benefits of the test outweigh the risk.[22]
With a mean radiation dose of 5.5 mSv, our study showed that a CTPA exam is associated with nearly 1.8 times the yearly background dose (equals 3 mSv)[26] an individual is exposed to in the US. This is much lower when compared to a prior study on older scanners, in which the mean dose was 9 mSv,[22] 3 times the background radiation dose. Even in our study, one of the older scanners (64SSwFBP) had a mean radiation dose of 9.29 mSv. Multivariate analysis showed that patient age >18 years, BMI >25 kg/m2, male sex, and ICU patients were independent determinants of increased radiation dose. BMI has been previously shown to have a linear association with DLP, effective mA, and effective dose.[27] In addition, BMI has been shown to have a good correlation with effective diameter in abdominal CT.[28] To the best of our knowledge, the relationship between radiation dose and BMI in patients getting CTPA exams has never been studied. The association of patient age >18 years and male sex with higher radiation dose is likely related to overall increased bodyweight and differences in the body-habitus. Prior studies have demonstrated higher radiation dose in males.[22] Males are, however, less radiosensitive and have lower cancer risk when exposed to similar (or even higher) radiation doses.[22] The specific reason for increased radiation dose to ICU patients was not studied but it was likely due to inability to raise their arms above the head in most cases, leading to increase CT tube output. This should be validated in future studies, to identify ways to decrease radiation dose in this cohort, which tends to have more imaging with higher positivity rates.[5]
Among CT scanner characteristics, our study showed that third generation dual source scanner (Siemens Somatom Force, Siemens Healthcare) with model-based Advanced Modeled IR (ADMIRE) was associated with significantly reduced doses (mean: 4.90 mSv). The scanner with older image reconstruction technique – FBP was associated with significantly higher radiation (mean: 9.29 mSv, P < 0.001) as compared to both single source and dual source scanner with IR. These findings are similar to prior studies that showed that IR techniques are associated with lower radiation dose and superior image quality as compared to FBP.[29-31] In a recent study, the use of advanced IR model was shown to have dose reduction up to 50% as compared to FBP.[15] However, the study does not mention the specific type of IR method used and the sample size was small (92 subjects). IR methods are also shown to be associated with higher contrast to noise ratio (CNR)[15,32] which allows for a decrease in the intravenous contrast dose as well. As IR images (especially at higher levels of IR) are subjectively rated as artificial and waxy when compared to FBP, low level (strength) of IR is preferred. Our study shows the combined effect of advances in CT technology and image reconstruction algorithm significantly reducing radiation dose in a large patient set. With technological advances, reduction in radiation dose is seen with CT evaluation of other vascular and non-vascular diseases.[33-37] Combined use of dual source scanner and third generation model-based IR (ADMIRE) decreased the radiation dose 54.3% as compared to 64 slice-single source scanner with FBP and by 18.4% as compared to 128 slice-single source scanner with first generation IR (SAFIRE). This highlights the dynamic evolution of reconstruction algorithms with higher reduction in radiation dose with advancing CT and IR techniques. Similar to our results, Yasaka et al.[38] also demonstrated greater reduction in radiation dose using model-based IR techniques compared to first generation statistical IR algorithms in patients undergoing abdominopelvic CTs. In addition, in our study, there was no significant difference in radiation doses between 128 slice-single source scanners with the first generation IR (128SSwSAFIRE) and 64 slice-single source scanners with first generation IR (64SSwSAFIRE). This suggests that the radiation dose decrease is more related to the generation of IR rather than the generation of CT scanner. Based on the results of the study, it would make more sense to scan ICU patients and those with high BMI, on the scanner that would have advanced IR image reconstruction technique to achieve maximum radiation dose reduction. These are also the patients that are more likely to have a positive exam[5] and hence a good-quality exam is crucial.
Limitations
The retrospective design and use of a single radiation dose conversion factor for patients across different age and sex are the primary limitations of this study. However, our sample was mainly adults with BMI >35 kg/m2 and hence this study is most applicable to this cohort. Relatively large sample size should also help to negate errors because of lack of individualized radiation dose calculation. The other limitation may be the use of effective dose estimation using k-conversion factor instead of size-specific dose estimate (SSDE), which takes into account the size of the patient. However, the SSDE calculation has its own drawbacks; SSDE requires measurement of anteroposterior and lateral diameters which is time-consuming and is prone to interobserver measurement variability.[28] Second, it is difficult to interpret and does not allow comparison with bench mark regulatory dose limits as SSDE does not allow calculation of effective dose. The concept of effective dose provides easy comparability among several diagnostic modalities. Another approach would have been to use tissue weighting factors; however, calculating individual organ doses for our large data set would have been extremely cumbersome. This also prevented us from calculating breast dose in female patients. We used the DLP in estimating effective dose as it is readily available on current scanners, can be easily converted to effective dose using k-conversion factors and more generalizable for use in a real world clinical setting.
As this study was performed in a single tertiary care center the results may not be applicable to smaller facilities or community hospital that does not have access to the latest generation of scanners/reconstruction software.
The strength of our study lies in large sample size and data form CTPA exams performed on different scanners on patients with differing demographics, using a uniform scanning protocol. This study design was adequate to answer the questions that we raised.
CONCLUSION
Male gender, patient age >35 years, patients in the ICU, and BMI >25 kg/m2 were the factors associated with significantly higher radiation dose. Among scanners, 64-slice single source scanners with FBP image reconstruction are associated with highest radiation dose and third generation dual source scanners with model-based IR are associated with least radiation dose. Patients with characteristics associated with the higher radiation dose (high BMI, ICU referrals, and male sex) would benefit from CTPA imaging on scanners with model-based IR, if available to prevent very high radiation dose.
Declaration of patient consent
Institutional Review Board permission obtained for the study.
Financial support and sponsorship
Nil.
Conflicts of interest
There are no conflicts of interest.
References
- National Hospital Ambulatory Medical Care Survey: 2016 Emergency Department Summary. 2016. Available from: https://www.cdc.gov/nchs/data/nhamcs/web_tables/2016_ed_web_tables.pdf [Last accessed on 2020 Aug 10]
- [Google Scholar]
- Trends in use and yield of chest computed tomography with angiography for diagnosis of pulmonary embolism in a Connecticut hospital emergency department. Conn Med. 2010;74:5-9.
- [Google Scholar]
- U.S. trends in computed tomography use and diagnoses in emergency department visits by patients with symptoms suggestive of pulmonary embolism 2001-2009. Acad Emerg Med. 2013;20:1033-40.
- [CrossRef] [PubMed] [Google Scholar]
- Pulmonary embolism imaging: When is it too much? Postgrad Med J. 2019;95:177.
- [CrossRef] [PubMed] [Google Scholar]
- Pulmonary embolism rule out: Positivity and factors affecting the yield of CT angiography. Postgrad Med J. 2020;96:594-9.
- [CrossRef] [PubMed] [Google Scholar]
- Overutilisation of imaging studies for diagnosis of pulmonary embolism: Are we following the guidelines? Postgrad Med J. 2019;95:420-4.
- [CrossRef] [PubMed] [Google Scholar]
- Inappropriate use of D-dimer assay and pulmonary CT angiography in the evaluation of suspected acute pulmonary embolism. Am J Med Qual. 2012;27:74-9.
- [CrossRef] [PubMed] [Google Scholar]
- Trends and variation in the utilization and diagnostic yield of chest imaging for medicare patients with suspected pulmonary embolism in the emergency department. AJR Am J Roentgenol. 2018;210:572-7.
- [CrossRef] [PubMed] [Google Scholar]
- CT pulmonary angiography: Increasingly diagnosing less severe pulmonary emboli. PLoS One. 2013;8:e65669.
- [CrossRef] [PubMed] [Google Scholar]
- Exposure to diagnostic radiation and risk of breast cancer among carriers of BRCA1/2 mutations: Retrospective cohort study (GENE-RAD-RISK) BMJ. 2012;345:e5660.
- [CrossRef] [PubMed] [Google Scholar]
- Radiation dose associated with common computed tomography examinations and the associated lifetime attributable risk of cancer. Arch Intern Med. 2009;169:2078-86.
- [CrossRef] [PubMed] [Google Scholar]
- International variation in radiation dose for computed tomography examinations: Prospective cohort study. BMJ. 2019;364:k4931.
- [CrossRef] [PubMed] [Google Scholar]
- Comparison of filtered back projection, hybrid iterative reconstruction, model-based iterative reconstruction, and virtual monoenergetic reconstruction images at both low-and standard-dose settings in measurement of emphysema volume and airway wall thickness: A CT phantom study. Korean J Radiol. 2018;19:809-17.
- [CrossRef] [PubMed] [Google Scholar]
- Ultra-low dose contrast CT pulmonary angiography in oncology patients using a high-pitch helical dual-source technology. Diagn Interv Radiol. 2019;25:195-203.
- [CrossRef] [PubMed] [Google Scholar]
- CT pulmonary angiography: Dose reduction via a next generation iterative reconstruction algorithm. Acta Radiol. 2019;60:478-87.
- [CrossRef] [PubMed] [Google Scholar]
- Sinogram-affirmed iterative reconstruction of low-dose chest CT: Effect on image quality and radiation dose. AJR Am J Roentgenol. 2013;201:W235-44.
- [CrossRef] [PubMed] [Google Scholar]
- A prospective evaluation of dose reduction and image quality in chest CT using adaptive statistical iterative reconstruction. AJR Am J Roentgenol. 2010;195:1095-9.
- [CrossRef] [Google Scholar]
- Multisection CT protocols: Sex-and age-specific conversion factors used to determine effective dose from dose-length product. Radiology. 2010;257:158-66.
- [CrossRef] [PubMed] [Google Scholar]
- Over-testing for suspected pulmonary embolism in American emergency departments: The continuing epidemic. Circ Cardiovasc Qual Outcomes. 2020;13:e005753.
- [CrossRef] [Google Scholar]
- “over-testing for suspected pulmonary embolism in American emergency departments: The continuing epidemic”. Circ Cardiovasc Qual Outcomes. 2020;13:e006560. regarding article
- [CrossRef] [PubMed] [Google Scholar]
- Overuse of computed tomography pulmonary angiography in the evaluation of patients with suspected pulmonary embolism in the emergency department. Acad Emerg Med. 2012;19:1219-26.
- [CrossRef] [PubMed] [Google Scholar]
- Risk-benefit analysis of pulmonary CT angiography in patients with suspected pulmonary embolus. AJR Am J Roentgenol. 2012;198:1332-9.
- [CrossRef] [PubMed] [Google Scholar]
- Prospective study of the incidence of contrast-induced nephropathy among patients evaluated for pulmonary embolism by contrast-enhanced computed tomography. Acad Emerg Med. 2012;19:618-25.
- [CrossRef] [PubMed] [Google Scholar]
- Relation of contrast nephropathy to adverse events in pulmonary emboli patients diagnosed with contrast CT. Am J Emerg Med. 2016;34:1247-50.
- [CrossRef] [Google Scholar]
- Use of intravenous iodinated contrast media in patients with kidney disease: Consensus statements from the American college of radiology and the national kidney foundation. Radiology. 2020;294:192094.
- [CrossRef] [PubMed] [Google Scholar]
- Radiation dose from medical imaging: A primer for emergency physicians. West J Emerg Med. 2012;13:202-10.
- [CrossRef] [PubMed] [Google Scholar]
- A new method for estimating of patient effective dose in computed tomography based on body mass index: Performance of the method in abdomen-pelvic examination. Iran J Med Phys. 2018;15:123.
- [Google Scholar]
- Using body mass index to estimate individualised patient radiation dose in abdominal computed tomography. Eur Radiol Exp. 2018;2:38.
- [CrossRef] [PubMed] [Google Scholar]
- Sinogram affirmed iterative reconstruction (SAFIRE) versus weighted filtered back projection (WFBP) effects on quantitative measure in the COPDGene 2 test object. Med Phys. 2014;41:091910.
- [CrossRef] [PubMed] [Google Scholar]
- Impact of advanced detector technology and iterative reconstruction on low-dose quantitative assessment of lung computed tomography density in a biological lung model. Med Phys. 2018;45:3657-70.
- [CrossRef] [PubMed] [Google Scholar]
- Comparison of low-and ultralow-dose computed tomography protocols for quantitative lung and airway assessment. Med Phys. 2017;44:4747-57.
- [CrossRef] [PubMed] [Google Scholar]
- Ultra low dose CT pulmonary angiography with iterative reconstruction. PLoS One. 2016;11:e0162716.
- [CrossRef] [PubMed] [Google Scholar]
- Imaging of the aortic root on high-pitch non-gated and ECG-gated CT: Awareness is the key! Insights Imaging. 2020;11:51.
- [CrossRef] [PubMed] [Google Scholar]
- CT and MR imaging of the upper extremity vasculature: Pearls, pitfalls, and challenges. Cardiovasc Diagn Ther. 2019;9:S152-73.
- [CrossRef] [PubMed] [Google Scholar]
- Advances in imaging and management trends of traumatic aortic injuries. Cardiovasc Intervent Radiol. 2017;40:643-54.
- [CrossRef] [PubMed] [Google Scholar]
- Modern imaging techniques: Applications in the management of acute aortic pathologies. Postgrad Med J. 2015;91:449-62.
- [CrossRef] [PubMed] [Google Scholar]
- Radiation dose reduction techniques for chest CT: Principles and clinical results. Eur J Radiol. 2019;111:93-103.
- [CrossRef] [PubMed] [Google Scholar]
- Model-based iterative reconstruction for reduction of radiation dose in abdominopelvic CT: Comparison to adaptive statistical iterative reconstruction. Springerplus. 2013;2:209.
- [CrossRef] [PubMed] [Google Scholar]